Abstract
Bipolar disorder is a psychiatric disorder characterized by recurrent episodes of mania/hypomania and depression. Progressive cognitive dysfunction such as impairments in executive function and verbal memory is common in euthymic bipolar patients. The cerebrospinal fluid has previously been used to study neurodegenerative processes in Alzheimer’s disease, from which changes in three core biomarkers have emerged as indicative of degeneration: amyloid β, total tau, and hyperphosphorylated tau. Here, neurodegeneration in bipolar disorder was investigated by assessing the association between bipolar disorder and cerebrospinal fluid biomarkers for neurodegenerative processes. Cerebrospinal fluid was obtained from 139 bipolar disorder patients and 71 healthy controls. Concentrations of total and phosphorylated tau, amyloid β1-42, amyloid β38/β40/β42, and the soluble forms of amyloid precursor protein were measured in patients vs controls. The concentrations of the soluble forms of amyloid precursor protein were significantly lower in bipolar patients compared with controls. The amyloid β42/amyloid β38 and the amyloid β42/amyloid β40 ratios were higher in bipolar patients than controls. There were no discernible differences in the concentrations of total/phosphorylated tau, amyloid β1-42, or amyloid β38/β40/β42. The concentrations of the biomarkers within the bipolar patient group were further associated with different ongoing medical treatments and diagnostic subgroups. The findings suggest that the amyloid precursor protein metabolism is altered in bipolar disorder. The results may have implications for the understanding of the pathophysiology of bipolar disorder and for the development of treatment strategies. Importantly, there were no signs of an Alzheimer-like neurodegenerative process among bipolar patients.
Similar content being viewed by others
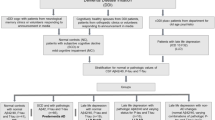
INTRODUCTION
Bipolar disorder is a common psychiatric disorder affecting about 1–3% of the population (Merikangas et al, 2011). It is characterized by recurrent episodes of mania (or hypomania) and depression, interspaced with periods of euthymic mood (Belmaker, 2004). In schizophrenia, associated cognitive impairment has been well known ever since the first description of the disease more than 100 years ago (initially described as dementia praecox) but only recently has the importance of cognitive dysfunction for bipolar disorder been fully addressed. Cognitive impairment in schizophrenia involves attention, speed of processing, working/long-term memory, executive function, and social cognition (Fioravanti et al, 2005). Accumulating evidence suggests similar but less pronounced cognitive impairment in bipolar disorder, including executive function and verbal memory (Martinez-Aran et al, 2000; Robinson et al, 2006). Indeed, cognitive impairment has been suggested to be one of the most important contributors to functional decline in bipolar disorder (Zarate et al, 2000; Sanchez-Moreno et al, 2009). Cognitive dysfunction progression has been further associated with number of manic episodes and the duration of illness (Martinez-Aran et al, 2004). Moreover, a range of neuromorphological magnetic resonance imaging studies have indicated that the decline in cognitive function is associated with structural brain changes, including enlargement of the lateral ventricles and deep white matter hyperintensities (Kempton et al, 2008; Konarski et al, 2008). We recently found that the number of manic episodes were strongly associated with gray matter reduction in the inferior frontal gyri of the dorsolateral prefrontal cortices (Ekman et al, 2010). In addition, it has been reported that psychiatric disorder might be a risk factor for late-life dementia (Cooper and Holmes, 1998). Taken together, these findings raise the question whether neurodegenerative processes are involved in the pathophysiology of bipolar disorder.
The cerebrospinal fluid (CSF) is a reservoir of potential biomarkers reflecting the biochemistry of the brain. CSF has previously successfully been used to study the pathophysiology of bipolar disorder (Ryden et al; 2009a Olsson et al, 2010) and has been extensively utilized in the quest to identify biomarkers in Alzheimer’s disease, whereby three core biomarkers have emerged: the 42 amino-acid isoform of amyloid β (Aβ42), total tau (T-tau), and hyperphosphorylated tau (P-tau) (Blennow et al, 2010). These biomarkers have been used to study pathophysiological features of Alzheimer’s disease, including amyloid deposition (Aβ42), axonal degradation (T-tau), and tangle formation (P-tau). High CSF concentrations of T-tau and P-tau together with low concentrations of Aβ42 are strongly correlated with the cognitive decline seen in Alzheimer’s disease patients (Blennow et al, 2010). Aβ42 is a product of enzymatic cleavages by β- and γ-secretase of amyloid precursor protein (APP). APP is a transmembrane protein of largely unknown function widely expressed in the brain. APP can also undergo non-amyloidogenic processing by α-secretase, which precludes formation of Aβ42 (Zhang et al, 2011). The α- or β-secretase cleavage of APP also generates soluble forms of APP called sAPP-α or sAPP-β, which can be measured in the CSF (Zhang et al, 2011). Furthermore, variation in γ-secretase cleavage generates differently sized Aβ (38–42 amino acids) (Schoonenboom et al, 2005). Tau is a neuronal protein localized to distal axons where it has been described to function as microtubule stabilizer (Morris et al, 2011). It is regulated by phosphorylation by kinases (eg, GSK-3β, CDK5, MARK, and MAPK), which is associated with disruption of microtubule organization (Morris et al, 2011). This is interesting given that GSK-3β (glycogen synthase kinase-3β) dysregulation has been suggested to have an important role in bipolar disorders and is a potential drug target to treat mood disorders (Jope, 2011). In addition to Alzheimer’s disease, abnormal CSF T-tau concentrations may be seen in other tauopathies like Pick’s disease, frontotemporal dementia, corticobasal degeneration, and progressive supranuclear palsy (Hernandez and Avila, 2007). T-tau and P-tau can also be detected in CSF from healthy individuals, and it is believed that variations within the normal concentration range reflect physiological synaptic and axonal turnover in the brain (Mattsson et al, 2010c).
Given the possible neurodegenerative component in the pathophysiology of bipolar disorder, we set out to investigate the CSF biomarkers known to be altered in Alzheimer’s disease. We used CSF from a large meticulously phenotyped patient–control study population that is adequately powered not only for studies of patient–control differences but also for the study of potential differences between subtypes of bipolar disorder and the role of medication.
SUBJECTS AND METHODS
Patients
Patients were recruited from the St Göran bipolar project, enrolling patients from the bipolar unit at the Northern Stockholm Psychiatric Clinic, Stockholm, Sweden. The work-up and diagnostic assessments have been described in detail previously (Ryden et al, 2009b). All patients were assessed by a psychiatrist (or psychiatrist in training) using a standardized interview protocol (the Affective disorders evaluation) previously used in the Systematic Treatment Enhancement Program of Bipolar Disorder program (Sachs et al, 2003). The Affective disorders evaluation guides the interviewer through a systematic assessment of the patient’s current mental state, past history, and diagnosis according to DSM-IV criteria as contained in the Structured Clinical Interview for DSM-IV (SCID). Co-morbid psychiatric disorders were screened for using Mini International Neuropsychiatric Interview (M.I.N.I.) (Sheehan et al, 1998). The full diagnostic assessment was based on all available sources of information, including patient interview, case records and, if possible, interviews with the next of kin. The diagnoses were set at a diagnostic case-conference where all information at the time of admission was presented. A consensus panel of experienced board-certified psychiatrists specialized in bipolar disorder (n=2–5) made a best-estimate diagnostic decision. Using this procedure, the risk of inter-rater bias in the inclusion process was reduced. The general criteria for inclusion were patients at least 18 years old and who met the DSM-IV criteria for any bipolar disorder, ie, type I, II, NOS (not otherwise specified), cyclothymia, or schizoaffective syndrome manic type. Information is collected about number of depressive, manic, and mixed episodes, history of suicide attempts, family history (first or second degree relatives with bipolar disorder), history of abuse (alcohol or substances), comorbid anxiety disorders (ie, panic disorder, social phobia, post-traumatic stress disorder, generalized anxiety disorder, obsessive-compulsive disorder, and agoraphobia), body mass index, and history of psychosis. The lifetime severity of bipolar disorder was rated using the Clinical Global Impression (CGI) rating scales. This seven-point scale reflects the clinician’s rate of the severity: 1=normal or not at all ill, 2=borderline mentally ill, 3=mildly ill, 4=moderately ill, 5=markedly ill, 6=severely ill, and 7=extremely ill. In order to determine euthymia, the Montgomery–Åsberg Depression Rating Scale (MADRS) and the young mania rating scale (YMRS) were used (euthymia defined as MADRS<14 and YMRS<14). For ethical reasons, patients continued to take their prescribed medications at the time of CSF sampling.
Age- and sex-matched healthy, population-based controls were randomly selected by Statistics Sweden (SCB) and contacted by mail. Interested persons contacted the study team that conducted a preliminary telephone screening to exclude severe mental health and neurological problems as well as substance abuse. Eligible persons were scheduled for a 1-day comprehensive assessment. Of the controls that received the invitation mail, 14% contacted the research team. This is on par with other studies of similar nature according to SCB. Of those controls that volunteered, 75 were excluded at the telephone interview mainly due to drug use (N=16), changed their mind (N=14), somatic ill-health (N=12), metal objects in body excluding magnetic resonance imaging (N=10), heredity in first-degree relative of bipolar disorder or schizophrenia (N=9), ongoing mental health diagnoses (N=6), pregnancy (N=5), and moved out of area (N=2). One subject had no documented reason for exclusion. Furthermore, one subject failed to show up for the assessments. Control subjects underwent a psychiatric interview by experienced clinicians using the M.I.N.I. to exclude psychiatric disorders (Sheehan et al, 1998). Moreover the controls completed the same investigations the patients had undertaken, including self-rating scales, somatic tests, blood tests, and lumbar puncture. Because the assessments of controls might reveal pathological findings, case conferences were held between examining clinicians, primary investigator, and the study coordinator to decide whether or not to include such persons in the study. It was thus decided to allow past minor depressive episodes, isolated episodes of panic disorder, eating disorders, or obsessive compulsive disorder that had remitted spontaneously or with brief psychotherapy counseling. Substance abuse was screened for at the telephone interview by the nurse, in the psychiatric interview, by AUDIT and DUDIT, as well as by determining serum levels of carbohydrate-deficient transferrin (Saunders et al, 1993). Overconsumption of alcohol as revealed by carbohydrate-deficient transferrin or responses indicating large consumption (>8 standard drinks per time more than 2 times per week) and/or amnesia and/or loss of control more than once per month resulted in the exclusion of these individuals from the study. Other exclusion criteria were neurological conditions other than mild migraines, untreated endocrinological disorders, pregnancy, dementia, recurrent depressive disorder, and suspected severe personality disorders (based on interview and SCID-II personality assessment) and a family history of schizophrenia or bipolar disorder in first-degree relatives.
The study was approved by the Regional Ethics Committee in Stockholm and conducted in accordance with the latest Helsinki Protocol. After complete description of the study, all enrolled patients and controls consented orally and in writing to participate in the study.
Neuropsychology Testing
The neuropsychological assessments included were Wechsler Adult Intelligence Scale (WAIS) version III (WAIS-III) and Trail making test (TMT). WAIS-III measures human intelligence and provides scores for both verbal intelligence quote (IQ) and a performance IQ. The WAIS-III also elicits four indices based on different sets of subtests: verbal comprehension, perceptual organization, working memory, and processing speed. The TMT consists of a visual cancellation task and a series of connect-the-circle tasks (part of Delis–Kaplan Executive Function System, D-KEFS). TMT involves five conditions, including visual scanning (TMT-1), number sequencing (TMT-2), letter sequencing (TMT-3), number-letter switching (TMT-4), and motor speed (TMT-5). The psychologists performing the neuropsychological assessments were not blind with respect to whether the test person was a patient or control.
CSF Sampling
CSF sampling (lumbar puncture) was performed when the participants were in a stable euthymic mood. Subjects fasted overnight before the CSF collection that occurred between 0900 and 1000 hrs. The spinal needle was inserted into the L3/L4 or L4/L5 interspace, and a total volume of 12 ml of the CSF was collected, gently inverted to avoid gradient effects, and divided into 1.0–1.6 ml aliquots that were stored at −80 °C pending analysis. An identical procedure was performed for the controls. All samples in this study were thawed and refrozen once before analysis. The staff performing the lumbar puncture was not blind with respect to whether the test person was a patient or control.
Biomarker Analyses
All biochemical analyses were performed at the Clinical Neurochemistry Laboratory in Mölndal, Sweden, by experienced and board-certified laboratory technicians who were blind with respect to the clinical information. The CSF concentrations of sAPP-α and sAPP-β, and Aβ38, Aβ40, and Aβ42 were determined using the MSD sAPP-α/sAPP-β Multiplex Assay and MSDHuman/Rodent (4G8) Abeta-Triplex Assay, respectively, as described by the manufacturer (Meso Scale Discovery, Gaithersburg, MD, USA). CSF concentrations of hyperphosphorylated-Tau (P-tau), total-tau (T-tau), and Aβ1-42 were measured simultaneously by the Luminex xMAP technology using the Inno-Bia AlzBio3 kit (Innogenetics, Zwijndrecht, Belgium). The inter-assay coefficient of variability was 8% (sAPP-α), 20% (sAPP-β), 2% (Aβ38), 15% (Aβ40), and 13% (Aβ42). The intra-assay coefficient of variability was <10% for all biomarkers.
Statistics
The statistics software package SPSS 19 (SPSS Inc, USA) was used for the statistical analysis. Pearson Chi-square test and Mann–Whitney test was used to analyze gender and age differences between patients and controls. T-test was used to analyze patient–control differences in neuropsychological test performance. Spearman’s Rho coefficient was used to analyze correlations between biomarker concentrations and neuropsychological test performance. Levene’s test for equality of variances was used for selection of appropriate T-test. Linear regression was used to analyze patient–control differences using gender and age as covariates. Multiple linear regression analysis (using stepwise method with criteria: probability-of-F-to-enter⩽0.050, probability-of-F-to-remove⩾0.100) was used to identify biomarker concentration predictors within the bipolar patient group. All p-values are presented as two-tailed and p-values <0.05 were regarded as significant. For patient–control comparisons, false discovery rate was used to correct for multiple comparisons and q-values <0.05 were regarded as significant.
RESULTS
Demographics and Medication
In this study, we included 139 bipolar patients and 71 healthy controls (45 females and 26 males). The median (interquartile) age of the controls was 32 (27–43) years. Demographics and clinical characteristics of the patient group are presented in Table 1. The patient group did not differ from the control group with respect to gender (χ2=0.172, df=1, p=0.678) or age (U(209)=5429.5, Z=1.19, p=0.234). A trend of higher body mass index was observed in patients with bipolar disorder (U(209)=5748.0, Z=−1.95, p=0.051). The prescription drugs were divided into the following major groups: lithium, lamotrigine, valproate, selective serotonin reuptake inhibitors, noradrenergic and specific serotonergic antidepressants, serotonin-norepinephrine reuptake inhibitors, benzodiazepines, and atypical antipsychotics. Patients were then classified based on use or non-use of medication from these groups at the time of CSF sampling (Table 1). Other medications not belonging to any of the major subgroups were clomipramine (N=4), nortriptyline (N=2), bupropion (N=2), reboxetine (N=1), moclobemide (N=1), hydroxyzine (N=1), levomepromazine (N=4), zuclopenthixol (N=2), haloperidol (N=1), and perphenazine (N=1). Many patients were on a combination of medications.
Table 2 shows the neuropsychological assessment scores (ie, WAIS-III and TMT) for the bipolar group and the healthy control group. The bipolar patients scored worse in several of the assessments, which is in line with previous studies of cognitive function in bipolar disorder (Martinez-Aran et al, 2000; Robinson et al, 2006).
Patient–Control Comparisons
The concentrations of seven different neurodegenerative biomarkers, including T-tau, P-tau, Aβ1-42, Aβ38, Aβ40, Aβ42, sAPP-α, and sAPP-β, were determined in the CSF from 139 patients and 71 healthy controls (Figure 1).
Grouped scatter plot showing the cerebrospinal fluid concentrations of T-tau, P-tau, Aβ1-42, Aβ38, Aβ40, Aβ42, sAPP-α, and sAPP-β in healthy controls (N=71) and bipolar patients (N=139). The median is shown as a straight line.
The concentrations of sAPP-α were significantly lower in bipolar patients when compared with controls (Table 3). A trend of low concentrations of sAPP-β was also observed (Table 3). There were no significant differences in CSF concentrations of T-tau, P-tau, Aβ1-42, Aβ38, Aβ40, or Aβ42 between the two groups (Table 3).
The ratios between the truncated forms of Aβ (ie, Aβ38 and Aβ40) and Aβ42 have been used for increasing the sensitivity of these biomarkers for Alzheimer’s disease. This is because decreased concentrations of Aβ42 in combination with higher concentrations of Aβ38 and Aβ40 have been observed in patients compared with controls (Schoonenboom et al, 2005). We found an increase in the Aβ42/Aβ38 in bipolar patients compared with controls (Table 3). A trend of increased Aβ42/Aβ40 ratio was also observed (Table 3).
Variables Predicting CSF Biomarker Concentrations in Bipolar Disorder Patients
In order to identify the possible explanatory variables for the CSF biomarker concentrations within the patient group, we conducted a post-hoc multiple linear regression analysis using the demographics and clinical characteristics outlined in Table 1 as independent variables. Patients with missing data were excluded, generating an inclusion of 128 patients for the regression analysis. The resulting regression models (using stepwise forward modeling) for each biomarker are shown in Table 4. In summary, the sAPP-α and sAPP-β concentrations were negatively associated with use of benzodiazepines, body mass index, and comorbid anxiety disorder as well as positively associated with use of NaSSA (ie, use of mirtazapine) and gender (males). The Aβ42/Aβ38 ratio was positively associated with use of valproate and negatively associated with lithium and age. The Aβ42/Aβ40 ratio was positively associated with bipolar type II and negatively associated with MADRS. The concentrations of Aβ peptides were positively associated with bipolar type II (Aβ42 and Aβ1-42) and negatively associated with disease severity (Aβ38 and Aβ40). No medication or clinical characteristic was significantly associated with either the T-tau or the P-tau concentrations (except for positive correlation between age and T-tau, data not shown).
We also analyzed the difference between healthy controls and bipolar type I patients and bipolar type II patients, respectively, for each biomarker (Supplementary Tables S1 and S2). In summary, the patient–control difference in sAPP-α and sAPP-β concentrations were significant only for bipolar type I patients, whereas the patient–control difference in Aβ42/Aβ40 and Aβ42/Aβ38 ratios were significant only for bipolar type II patients.
Correlations Between Neuropsychological Test Performance and CSF Biomarker Concentrations
We next examined whether there were any associations between biomarker concentrations and neurocognitive performance in patients and healthy controls (Supplementary Table S3 and S4). After correction of multiple testing, we found that the TMT-3 test was significantly positively associated with the concentrations of sAPP-α, sAPP-β, Aβ40, and T-tau. At a trend level, TMT-3 was also positively associated with Aβ38, Aβ42, and Aβ1-42. T-tau was also positively associated with TMT-1, TMT-2, and working memory. No significant association was observed between biomarker concentrations and neurocognitive performance in healthy controls.
DISCUSSION
We analyzed CSF biomarkers for synaptic dysfunction and neurodegeneration in a large sample of bipolar disorder patients and healthy controls.
The most striking finding was lower concentrations of the soluble forms of APP (α and β) in bipolar patients compared with healthy controls. Subsequent statistical analysis indicated that this finding was most prominent among bipolar type I patients. It cannot be deduced from these results whether decreased APP expression, processing, or clearance of sAPP causes the decreased CSF sAPP concentrations. Low CSF concentrations of sAPP have been observed in other conditions, such as systemic lupus erythematosus, multiple sclerosis, and Lyme neuroborreliosis (Mattsson et al, 2010b, 2010a). These disorders are all neuroinflammatory, which might indicate a possible role of inflammation in the pathogenesis of bipolar disorder. By contrast, high plasma concentrations of sAPP-α have been reported in autistic patients (Bailey et al, 2008; Ray et al, 2011). In Alzheimer’s disease, both unaltered and elevated concentrations of sAPP-α and sAPP-β have been reported (Lewczuk et al, 2010; Perneczky et al, 2011; Rosen et al, 2012).
The function of the soluble fragments of APP has been extensively evaluated in experimental studies, pointing to a neurotrophic role of secreted APP (Turner et al, 2003). For instance, sAPP has been shown to stimulate stem cell proliferation/differentiation/migration, stimulate synaptogenesis, protect neurons, and modulate synaptic plasticity/learning/memory (Turner et al, 2003). One might therefore speculate that the low CSF concentration of soluble APP in bipolar disorder reflects decreased neuronal/synaptic regenerative capacity, plasticity, and survival, which may, in turn, impact on cognitive functioning. Reduced concentrations of other neurotrophic factors (eg, brain-derived neurotrophic factor) have indeed been observed in bipolar disorder (Cunha et al, 2006), supporting a role of neurotrophins in the pathophysiology. Interestingly, APP has also been functionally linked to Disrupted-in-Scizhophrenia 1, a risk factor for bipolar disorder and other mental illnesses (Young-Pearse et al, 2010; Bradshaw and Porteous, 2012).
Low sAPP-α and sAPP-β concentrations were associated with benzodiazepine use in this study. It is possible that benzodiazepines decrease the expression of soluble APP, but another possibility is that low CSF concentrations of soluble APP are associated with a certain type of bipolar patients who require benzodiazepine treatment, eg, those with a comorbid anxiety disorder. Indeed, the concentrations of both sAPP-α and sAPP-β were negatively associated with comorbid anxiety. Likewise, the positive association with NaSSA treatment is difficult to interpret in this cross-sectional design.
A second finding was increased Aβ42/Aβ38 and Aβ42/Aβ40 ratios in the bipolar group compared with controls, despite no observable differences with respect to full length or truncated forms of Aβ peptide concentrations. This should be compared with previously observed low ratios in neurodegenerative disorders (Schoonenboom et al, 2005) and argues against neurodegenerative processes of Alzheimer’s type in bipolar disorder. However, increased Aβ42/Aβ38 and Aβ42/Aβ40 ratios have been suggested to be neurotoxic before plaque deposition in Alzheimer’s disease (ie, Aβ42 is the most toxic amyloid β peptide) (Jacobsen et al, 2006; Kuperstein et al, 2010). It is therefore possible that the increased ratio in bipolar patients indicates a precursor state involving amyloid β. The specific association between high concentrations of Aβ42 and bipolar type II patients further indicates that this finding is more related to depression than mania.
The use of valproate was associated with a high Aβ42/Aβ38 ratio and the use of lithium associated with a low Aβ42/Aβ38 ratio in this study. Interestingly, this is in line with previous reports that valproate and lithium interferes with APP cleavage at the γ-secretase step (Phiel et al, 2003; Qing et al, 2008). A possible interpretation of these findings is thus that the observed patient–control differences in Aβ42/Aβ38 ratio reflect a general change in γ-secretase activity in bipolar disorder. However, whether this effect of valproate or lithium treatment is related to its therapeutic effect in bipolar disorder is unknown. Valproate is generally used as an alternative to lithium treatment (eg, only 1 out of 17 valproate-taking patients in this study were also taking lithium). Thus, it is possible that these finding indicates a biological difference between lithium and valproate responders.
We also found that the total concentrations of the different Aβ peptides were generally lower in type I than in type II bipolar patients (mainly Aβ42 and Aβ1-42). The Aβ peptides (mainly Aβ38 and Aβ40) were further negatively associated with disease severity (as measured with CGI score). Thus, it is possible that in patients with severe forms of bipolar disorder, an Alzheimer-like decrease of CSF Aβ concentrations is present. For comparison, cognitive dysfunctions have indeed been shown to be associated with a progress of the course and severity of the disease (Cavanagh et al, 2002). In addition, low concentrations of Aβ42 and Aβ40 have been reported in patients with severe autism (Ray et al, 2011), major depression (Pomara et al, 2012), and in elderly schizophrenia patients (Frisoni et al, 2011), indicating similar mechanisms in these disorders.
If bipolar disorder would be associated with a neurodegeneration of Alzheimer’s type, we would expect a decrease of Aβ42—reflecting Aβ accumulation in the brain—in conjunction with increased T-tau and P-tau concentrations (Blennow et al, 2010). We found, however, no such changes in bipolar patients compared with healthy controls. This conclusion is in accordance with a previous study where no increase of amyloid plaques and neurofibrillary tangles were found in post-mortem brain tissues from psychiatric patients (Damadzic et al, 2002). However, Aβ42 and tau are only biomarkers for accumulation of amyloid plaques and neurofibrillary tangles; other neurodegenerative processes cannot be excluded. Indeed, many other components have been suggested to drive progressive brain changes in bipolar disorder, including the dopaminergic system, inflammatory cytokines, oxidative stress, mitochondrial stress, and alterations in neurotrophic signaling (Berk et al, 2011). It should also be noted that the median age of this sample population was 36 years and that it is possible that differences in Aβ42 and tau might be observed in an older cohort of bipolar patients. It should be noted that the patients in the current study is followed up annually and that cognitive impairments in relation to neurochemical markers may by studied in the future.
Post-hoc, we analyzed possible correlations between biomarker concentrations and performance scores in neuropsychological tests (WAIS-III and TMT). No correlations were observed in healthy controls. In the bipolar patient group, by contrast, TMT-3 was positively associated to several biomarkers (including sAPP-α, sAPP-β, amyloid β, and T-tau). These findings hint at the possibility that these biomarkers are coupled to specific cognitive dysfunctions that might occur in patients with bipolar disorder. To explore this tentative link, however, further studies specifically addressing this question are warranted.
An important strength of this study is that CSF was collected from a large group of well-defined bipolar patients and a population-based control group. This provided us with sufficient power to detect differences and also to exclude neurodegeneration of Alzheimer’s type. Moreover, patients as well as controls were tested with a neuropsychological battery that demonstrated cognitive deficits in the patient group that were similar to previous findings. Hence, the findings in this study are likely to be generalizable.
It is important to note that this study merely provides a snapshot of the biomarker concentrations during the euthymic period of the disorder (trait marker). Changes in biomarker concentrations might be more apparent during manic and depressive episodes (state marker). However, it may also be argued that measuring biomarkers during euthymia provides a more reliable picture of bipolar disorder biomarker concentrations as these might be affected by secondary effects that appear during mania/depression (like altered sleeping behavior, motor activity, etc.) not specific to bipolar disorder. A limitation of this study is that, even though we adjusted for single-medication class use in the statistical analyses, there is a risk that either a combination of medications or previous drug intake might influence biomarkers concentrations. Another limitation of this study was that no correction for multiple comparisons was performed in the post-hoc multiple linear regression analyses within the patient group and that this can lead to a type I error, ie, false-positive findings. However, as we think that these findings suggest important directions for future clinical and experimental studies, we chose to report original p-values but stress that these findings should be interpreted cautiously. In any case, it is important to verify the current findings in an independent sample population to definitely rule out false-positive or false-negative associations.
In conclusion, this is the first study of biomarkers for neurodegeneration of Alzheimer type in bipolar disorder. We found that the concentrations of the soluble forms of APP were decreased in bipolar patients compared with healthy controls. These results suggest a possible role of neuroinflammation or neurotrophins in the pathogenesis of bipolar disorder. Further studies, including biomarkers for inflammation and neuropeptides, are warranted to further investigate this. The association between low concentration of Aβ with increasing disease severity and bipolar type I further supports that processes involving Aβ metabolism is involved in bipolar disorder. Importantly, we found no evidence of neurodegeneration of Alzheimer’s type in bipolar patients.
References
Bailey AR, Giunta BN, Obregon D, Nikolic WV, Tian J, Sanberg CD et al (2008). Peripheral biomarkers in Autism: secreted amyloid precursor protein-alpha as a probable key player in early diagnosis. Int J Clin Exp Med 1: 338–344.
Belmaker RH (2004). Bipolar disorder. N Engl J Med 351: 476–486.
Berk M, Kapczinski F, Andreazza AC, Dean OM, Giorlando F, Maes M et al (2011). Pathways underlying neuroprogression in bipolar disorder: focus on inflammation, oxidative stress and neurotrophic factors. Neurosci Biobehav Rev 35: 804–817.
Blennow K, Hampel H, Weiner M, Zetterberg H (2010). Cerebrospinal fluid and plasma biomarkers in Alzheimer disease. Nat Rev Neurol 6: 131–144.
Bradshaw NJ, Porteous DJ (2012). DISC1-binding proteins in neural development, signalling and schizophrenia. Neuropharmacology 62: 1230–1241.
Cavanagh JT, Van Beck M, Muir W, Blackwood DH (2002). Case-control study of neurocognitive function in euthymic patients with bipolar disorder: an association with mania. Br J Psychiatry 180: 320–326.
Cooper B, Holmes C (1998). Previous psychiatric history as a risk factor for late-life dementia: a population-based case-control study. Age Ageing 27: 181–188.
Cunha AB, Frey BN, Andreazza AC, Goi JD, Rosa AR, Goncalves CA et al (2006). Serum brain-derived neurotrophic factor is decreased in bipolar disorder during depressive and manic episodes. Neurosci Lett 398 215–219.
Damadzic R, Shuangshoti S, Giblen G, Herman MM (2002). Neuritic pathology is lacking in the entorhinal cortex, subiculum and hippocampus in middle-aged adults with schizophrenia, bipolar disorder or unipolar depression. Acta Neuropathol 103: 488–494.
Ekman CJ, Lind J, Ryden E, Ingvar M, Landen M (2010). Manic episodes are associated with grey matter volume reduction - a voxel-based morphometry brain analysis. Acta Psychiatr Scand 122: 507–515.
Fioravanti M, Carlone O, Vitale B, Cinti ME, Clare L (2005). A meta-analysis of cognitive deficits in adults with a diagnosis of schizophrenia. Neuropsychol Rev 15: 73–95.
Frisoni GB, Prestia A, Geroldi C, Adorni A, Ghidoni R, Amicucci G et al (2011). Alzheimer's CSF markers in older schizophrenia patients. Int J Geriatr Psychiatry 26: 640–648.
Hernandez F, Avila J (2007). Tauopathies. Cell Mol Life Sci 64: 2219–2233.
Jacobsen JS, Wu CC, Redwine JM, Comery TA, Arias R, Bowlby M et al (2006). Early-onset behavioral and synaptic deficits in a mouse model of Alzheimer's disease. Proc Natl Acad Sci USA 103: 5161–5166.
Jope RS (2011). Glycogen synthase kinase-3 in the etiology and treatment of mood disorders. Front Mol Neurosci 4: 16.
Kempton MJ, Geddes JR, Ettinger U, Williams SC, Grasby PM (2008). Meta-analysis, database, and meta-regression of 98 structural imaging studies in bipolar disorder. Arch Gen Psychiatry 65: 1017–1032.
Konarski JZ, McIntyre RS, Kennedy SH, Rafi-Tari S, Soczynska JK, Ketter TA (2008). Volumetric neuroimaging investigations in mood disorders: bipolar disorder versus major depressive disorder. Bipolar Disord 10: 1–37.
Kuperstein I, Broersen K, Benilova I, Rozenski J, Jonckheere W, Debulpaep M et al (2010). Neurotoxicity of Alzheimer’s disease Abeta peptides is induced by small changes in the Abeta42 to Abeta40 ratio. EMBO J 29: 3408–3420.
Lewczuk P, Kamrowski-Kruck H, Peters O, Heuser I, Jessen F, Popp J et al (2010). Soluble amyloid precursor proteins in the cerebrospinal fluid as novel potential biomarkers of Alzheimer’s disease: a multicenter study. Mol Psychiatry 15: 138–145.
Martinez-Aran A, Vieta E, Colom F, Reinares M, Benabarre A, Gasto C et al (2000). Cognitive dysfunctions in bipolar disorder: evidence of neuropsychological disturbances. Psychother Psychosom 69: 2–18.
Martinez-Aran A, Vieta E, Colom F, Torrent C, Reinares M, Goikolea JM et al (2005). Do cognitive complaints in euthymic bipolar patients reflect objective cognitive impairment? Psychother Psychosom 74: 295–302.
Martinez-Aran A, Vieta E, Reinares M, Colom F, Torrent C, Sanchez-Moreno J et al (2004). Cognitive function across manic or hypomanic, depressed, and euthymic states in bipolar disorder. Am J Psychiatry 161: 262–270.
Mattsson N, Bremell D, Anckarsater R, Blennow K, Anckarsater H, Zetterberg H et al (2010a). Neuroinflammation in Lyme neuroborreliosis affects amyloid metabolism. BMC Neurol 10: 51.
Mattsson N, Bremell D, Anckarsater R, Blennow K, Anckarsater H, Zetterberg H et al (2010b). Neuroinflammation in Lyme neuroborreliosis affects amyloid metabolism. BMC Neurol 10: 51.
Mattsson N, Savman K, Osterlundh G, Blennow K, Zetterberg H (2010c). Converging molecular pathways in human neural development and degeneration. Neurosci Res 66: 330–332.
Merikangas KR, Jin R, He JP, Kessler RC, Lee S, Sampson NA et al (2011). Prevalence and correlates of bipolar spectrum disorder in the world mental health survey initiative. Arch Gen Psychiatry 68: 241–251.
Morris M, Maeda S, Vossel K, Mucke L (2011). The many faces of tau. Neuron 70: 410–426.
Olsson SK, Samuelsson M, Saetre P, Lindstrom L, Jonsson EG, Nordin C et al (2010). Elevated levels of kynurenic acid in the cerebrospinal fluid of patients with bipolar disorder. J Psychiatry Neurosci 35: 195–199.
Perneczky R, Tsolakidou A, Arnold A, Diehl-Schmid J, Grimmer T, Forstl H et al (2011). CSF soluble amyloid precursor proteins in the diagnosis of incipient Alzheimer disease. Neurology 77: 35–38.
Phiel CJ, Wilson CA, Lee VM, Klein PS (2003). GSK-3alpha regulates production of Alzheimer's disease amyloid-beta peptides. Nature 423: 435–439.
Pomara N, Bruno D, Sarreal AS, Hernando RT, Nierenberg J, Petkova E et al (2012). Lower CSF Amyloid Beta Peptides and Higher F2-Isoprostanes in Cognitively Intact Elderly Individuals With Major Depressive Disorder. Am J Psychiatry 169: 523–530.
Qing H, He G, Ly PT, Fox CJ, Staufenbiel M, Cai F et al (2008). Valproic acid inhibits Abeta production, neuritic plaque formation, and behavioral deficits in Alzheimer’s disease mouse models. J Exp Med 205: 2781–2789.
Ray B, Long JM, Sokol DK, Lahiri DK (2011). Increased secreted amyloid precursor protein-alpha (sAPPalpha) in severe autism: proposal of a specific, anabolic pathway and putative biomarker. PLoS One 6: e20405.
Robinson LJ, Thompson JM, Gallagher P, Goswami U, Young AH, Ferrier IN et al (2006). A meta-analysis of cognitive deficits in euthymic patients with bipolar disorder. J Affect Disord 93: 105–115.
Rosen C, Andreasson U, Mattsson N, Marcusson J, Minthon L, Andreasen N et al (2012). Cerebrospinal fluid profiles of amyloid beta-related biomarkers in Alzheimer's disease. Neuromolecular Med 14: 65–73.
Ryden E, Johansson C, Blennow K, Landen M (2009a). Lower CSF HVA and 5-HIAA in bipolar disorder type 1 with a history of childhood ADHD. J Neural Transm 116: 1667–1674.
Ryden E, Thase ME, Straht D, Aberg-Wistedt A, Bejerot S, Landen M (2009b). A history of childhood attention-deficit hyperactivity disorder (ADHD) impacts clinical outcome in adult bipolar patients regardless of current ADHD. Acta Psychiatr Scand 120: 239–246.
Sachs GS, Thase ME, Otto MW, Bauer M, Miklowitz D, Wisniewski SR et al (2003). Rationale, design, and methods of the systematic treatment enhancement program for bipolar disorder (STEP-BD). Biol Psychiatry 53: 1028–1042.
Sanchez-Moreno J, Martinez-Aran A, Colom F, Scott J, Tabares-Seisdedos R, Sugranyes G et al (2009). Neurocognitive dysfunctions in euthymic bipolar patients with and without prior history of alcohol use. J Clin Psychiatry 70: 1120–1127.
Saunders JB, Aasland OG, Babor TF, de la Fuente JR, Grant M (1993). Development of the Alcohol Use Disorders Identification Test (AUDIT): WHO Collaborative Project on Early Detection of Persons with Harmful Alcohol Consumption--II. Addiction 88: 791–804.
Schoonenboom NS, Mulder C, Van Kamp GJ, Mehta SP, Scheltens P, Blankenstein MA et al (2005). Amyloid beta 38, 40, and 42 species in cerebrospinal fluid: more of the same? Ann Neurol 58: 139–142.
Sheehan DV, Lecrubier Y, Sheehan KH, Amorim P, Janavs J, Weiller E et al (1998). The Mini-International Neuropsychiatric Interview (M.I.N.I.): the development and validation of a structured diagnostic psychiatric interview for DSM-IV and ICD-10. J Clin Psychiatry 59 (Suppl 20): 22–33 quiz 34-57.
Turner PR, O’Connor K, Tate WP, Abraham WC (2003). Roles of amyloid precursor protein and its fragments in regulating neural activity, plasticity and memory. Prog Neurobiol 70: 1–32.
Young-Pearse TL, Suth S, Luth ES, Sawa A, Selkoe DJ (2010). Biochemical and functional interaction of disrupted-in-schizophrenia 1 and amyloid precursor protein regulates neuronal migration during mammalian cortical development. J Neurosci 30: 10431–10440.
Zarate CA, Tohen M, Land M, Cavanagh S (2000). Functional impairment and cognition in bipolar disorder. Psychiatr Q 71: 309–329.
Zhang YW, Thompson R, Zhang H, Xu H (2011). APP processing in Alzheimer's disease. Mol Brain 4: 3.
Acknowledgements
We thank Åsa Källén, Monica Christiansson, and Dzemila Secic for excellent technical assistance, the staff at the St Görans Bipolar Affective Disorder unit, including coordinator Martina Wennberg, study nurse Agneta Carlswärd-Kjellin, and data manager Haydeh Olofsson for the diagnostic assessments and enrolling patients for this study. Yngve Hallström is acknowledged for performing lumbar punctures on patients and controls. Erik Joas and Kristoffer Bäckman are acknowledged for statistical support and Cynthia Soderblom for comments on the manuscript. We also thank the patients and controls participating in this study. Financial support was provided through the regional agreement on medical training and clinical research between Stockholm County Council and the Karolinska Institutet (ALF 20100305); and through grants from the Swedish Medical Research Council (K2008-62x-14647-06-3, K2010-61X-21569-01-1, and K2010-61P-21568-01-4), the Brain foundation, the Söderberg Foundation, Alzheimer’s Association, and the Swedish foundation for Strategic research.
Author information
Authors and Affiliations
Corresponding author
Ethics declarations
Competing interests
ML declares that, over the past three years, he has received compensation for lectures from AstraZeneca, Lundbeck pharmaceuticals, Eli Lilly Sweden, Wyeth, Servier Sweden, and served at advisory board for AstraZeneca and Lundbeck pharmaceuticals. He has no other equity ownership, profit-sharing agreements, royalties, or patent. CJE has once received remuneration from Lundbeck for lecturing. Dr Blennow has served at Advisory Boards for Innogenetics, Belgium and Roche Diagnostics, Germany. All the other authors declare no conflict of interest.
Additional information
Supplementary Information accompanies the paper on the Neuropsychopharmacology website
Supplementary information
PowerPoint slides
Rights and permissions
About this article
Cite this article
Jakobsson, J., Zetterberg, H., Blennow, K. et al. Altered Concentrations of Amyloid Precursor Protein Metabolites in the Cerebrospinal Fluid of Patients with Bipolar Disorder. Neuropsychopharmacol 38, 664–672 (2013). https://doi.org/10.1038/npp.2012.231
Received:
Revised:
Accepted:
Published:
Issue Date:
DOI: https://doi.org/10.1038/npp.2012.231
Keywords
This article is cited by
-
Dementia risk in middle-aged patients with schizophrenia, bipolar disorder, and major depressive disorder: a cohort study of 84,824 subjects
European Archives of Psychiatry and Clinical Neuroscience (2023)
-
Cerebrospinal fluid proteomic study of two bipolar disorder cohorts
Molecular Psychiatry (2022)
-
A serum proteomic study of two case-control cohorts identifies novel biomarkers for bipolar disorder
Translational Psychiatry (2022)
-
Cerebrospinal fluid proteomics targeted for central nervous system processes in bipolar disorder
Molecular Psychiatry (2021)
-
Genome-wide study of immune biomarkers in cerebrospinal fluid and serum from patients with bipolar disorder and controls
Translational Psychiatry (2020)